28 July 2022
A Nanokelvin Microwave Freezer for Molecules
Researchers at the Max Planck Institute of Quantum Optics have developed a new method to cool gases of polar molecules to near absolute zero. This paves the way for studying quantum effects of exotic forms of matter.
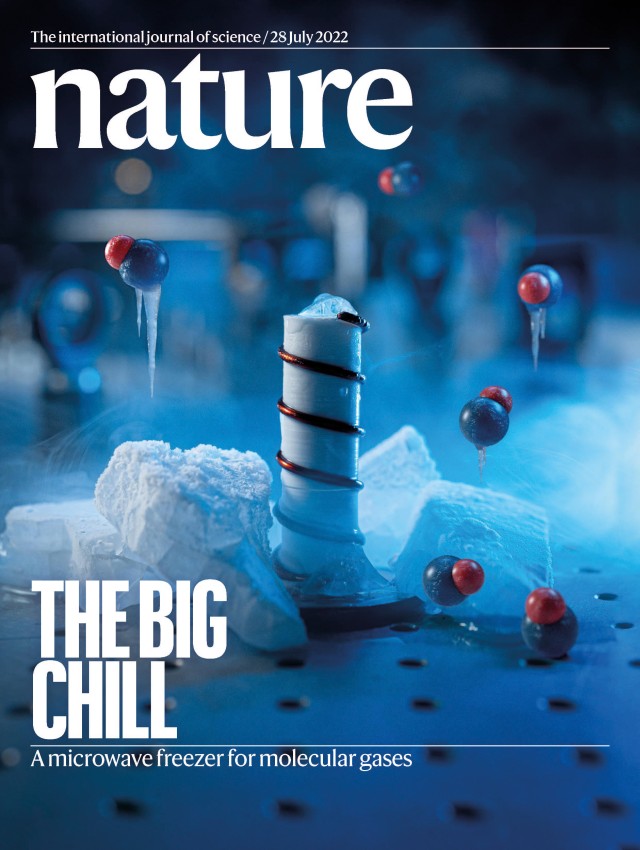
When a highly diluted gas is cooled to extremely low temperatures, bizarre properties are revealed. Thus, some gases form a so-called Bose-Einstein condensate - a type of matter in which all atoms move in unison. Another example is supersolidity: a state in which matter behaves like a frictionless fluid with a periodic structure. Physicists expect to find particularly diverse and revealing forms of quantum matter when cooling gases consisting of polar molecules. They are characterised by an uneven electrical charge distribution. Unlike free atoms, they can rotate, vibrate and attract or repel each other. However, it is difficult to cool molecular gases to ultra-low temperatures. A team of researchers at the Max Planck Institute of Quantum Optics (MPQ) in Garching has now found a simple and effective way to overcome this roadblock. It is based on a rotating field of microwaves.
A process like in a cup of coffee
For their experiments, the researchers used a gas of sodium-potassium (NaK) molecules that were confined in an optical trap by laser light. To cool the gas, the team relied on a method that has long proven effective for cooling unbound atoms: so-called evaporative cooling. "This method works similar to the familiar process, which causes a cup of hot coffee to cool down," says Dr. Xin-Yu Luo, head of the Laboratory for Ultracold Polar Molecules in the Division of Quantum Many-Body Systems at the MPQ: In coffee, water molecules constantly collide and thereby exchange parts of their kinetic energy. If two particularly energetic molecules collide, one of them can become fast enough to escape the coffee - it steams out of the cup. The other molecule remains with less energy. This is how the coffee gradually cools down. In the same way, a gas can be cooled down to a few nanokelvin - billionths of a degree above absolute zero at minus 273.15 degrees Celsius.
However: "If the gas consists of molecules, these must be additionally stabilised at very low temperatures," says Luo. The reason lies in the much more complex structure of molecules compared to unbound atoms. Therefore, controlling their movements during collisions is difficult. The molecules can stick together during collisions. Furthermore, "polar molecules behave like tiny magnets that can snap together, in which case they are lost for the experiment", explains Dr Andreas Schindewolf, who conducts research in Xin-Yu Luo's team. These difficulties have proven to be a huge roadblock to research in recent years.
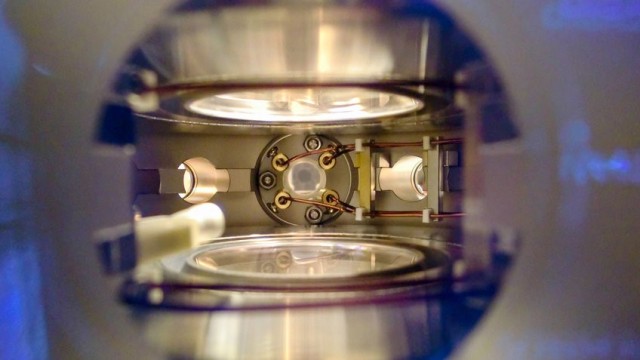
Microwaves keep the molecules apart
To overcome this obstacle, the researchers in Garching relied on a trick: the additional application of a specially prepared electromagnetic field that serves as an energetic shield for the molecules - preventing them from getting sticking together. "We created this energy shield using a strong, rotating microwave field," explains Andreas Schindewolf. "The field causes the molecules to rotate at a higher frequency." If two molecules come too close to each other, they can therefore exchange kinetic energy - but at the same time they align themselves in such a way that they repel each other and quickly seperate again.
To create a microwave field with the required properties, the researchers placed a helical antenna under the optical trap containing the gas of sodium-potassium molecules. "The rate at which the molecules became interlocked was thus reduced by more than one order of magnitude," reports Xin-Yu Luo. In addition, under the influence of the field, a strong and long-range electrical interaction developed between the molecules. "As a result, they collided much more frequently than without the rotating microwave field - on average about 500 times per molecule," says the physicist. "That was enough to cool the gas close to absolute zero through evaporation."
A new low-temperature record
After just a third of a second, the temperature reached around 21 nanokelvin - well below the critical "Fermi temperature". It marks the limit, below which quantum effects dominate the behaviour of a gas - and bizarre phenomena start to arise. "The temperature we have reached is the lowest so far in a gas of polar molecules," Luo is pleased to say. And the researcher believes that they can reach even far lower temperatures through technical refinements to the experimental setup.
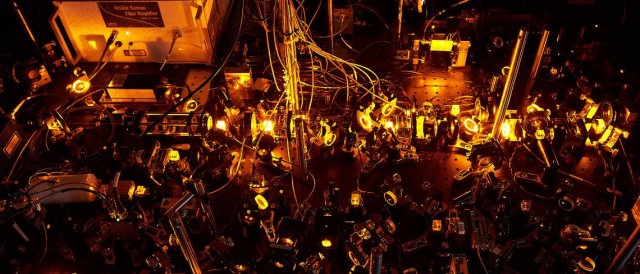
Source: MPQ Website
Publication
Evaporation of microwave-shielded polar molecules to quantum degeneracy
A. Schindewolf, R.Bause, X.-Y. Chen, M. Duda, T. Karman, I. Bloch & X.-Y. Luo
Nature 607, pages 677–681 (2022)
DOI:
10.1038/s41586-022-04900-0
Further reading
Research Briefing in Nature:
Molecules cooled in a microwave freezer
Contact
Dr. Andreas Schindewolf
Postdoc | Research Group "Ultracold Polar Molecules"
Max Planck Institute for Quantum Optics
andreas.schindewolf[at]mpq.mpg.de
Dr. Xinyu Luo
Research Group Leader | "Ultracold Polar Molecules"
Max Planck Institute for Quantum Optics
xinyu.luo[at]mpq.mpg.de
Katharina Jarrah
PR and Communications
Max Planck Institute of Quantum Optics
katharina.jarrah[at]mpq.mpg.de