16 April 2015
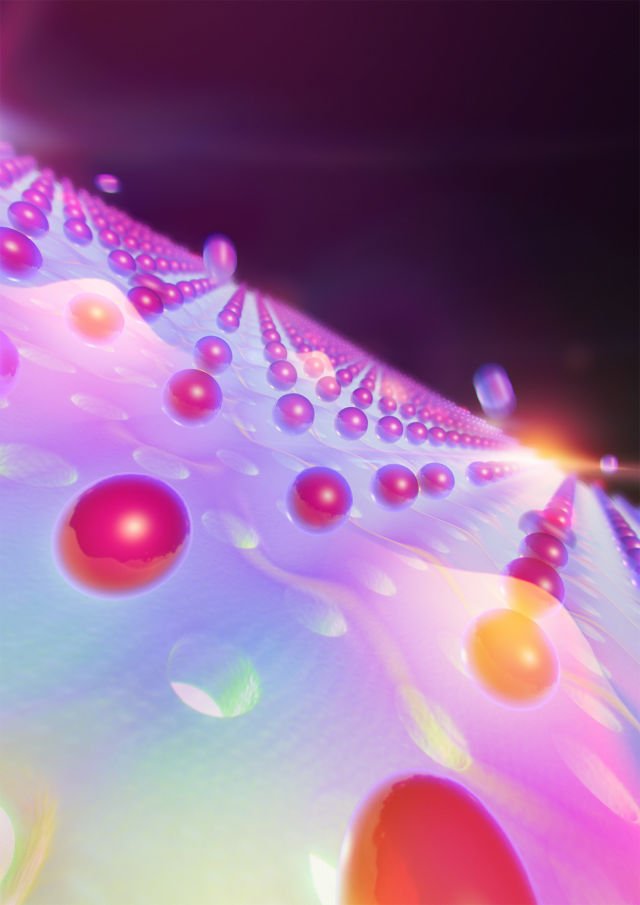
Ultracold atoms in the so-called optical lattices, that are generated by crosswise superposition of laser beams, have been proven to be one of the most promising tools for simulating and understanding the behaviour of many-body systems such as solid crystals – for example with respect to their electric or magnetic properties. However, the implementation in free space has some limitations such as the distance between the atoms (around 400 nm) and the short range of the interactions. Now a team of theorists around Prof Ignacio Cirac (MPQ) and
Prof. Jeff Kimble (Caltech) suggests a new set-up that integrates the advantages of ultracold atomic physics and nano-photonics to circumvent these limitations predicting lattice constants about ten times smaller than in a free space optical lattices and the possibility to mediate longer range interactions (Nature Photonics, AOP, 6 April 2015). The authors use the opportunities provided by nano-engineered dieletrics, the so-called Photonic Crystals, to study both how to trap the atoms closer to each other and make them interact through the guided modes in the structure. As a consequence, the energy scales of the system are increased as well as the range of the interactions, being able to explore new forms of quantum many-body matter.
The basic idea is to take a thin dielectric slab the refractive index of which gets periodically modulated by either drilling holes or installing little cylindrical posts in a grid-wise pattern. By using a combination between optical and vacuum forces, the authors show how to make lattices with up to 50 nm, around ten times smaller than for optical lattices.
“With these subwavelength lattices we can investigate about the same quantum many-body phenomena as in free space optical lattices,” explains Dr. Alejandro González-Tudela, a scientist in the Theory Division of Prof. Cirac and first author of the publication.
“But the difference and advantage of our proposed scheme is that the atoms are much closer to each other. That way we achieve higher tunneling rates and interaction energies for simulations of quantum many-body systems. And this implies that we can relax the cooling requirements of the atoms.” But it is not only the smaller scale of the lattice which provides the possibility to do new kinds of physics. The geometry of the two-dimensional thin dielectric layer allows trapping and guiding the light that falls onto the slab. So an incoming photon interacts strongly with an atom, and then it bounces off. But it does not fly into space: the photon propagates through the waveguide and finds another atom to interact with, and then it goes to the next one and interacts.
“Our analysis show that we should be able to achieve atom-atom-interactions, where the interaction mechanism is not by atom hopping (as in free space optical lattices) but by exchange of photons”, Alejandro González-Tudela says. “The result is a two-dimensional solid where the atoms are held together and talk to each other not by phonons – as in regular matter – but by photons. This implies a qualitatively new domain for light-matter interactions, with the capability to ‘design’ the strength and the range of the interactions. We would gain access to a rich set of phenomena, including, for example, quantum magnetism or spin-spin-interactions mediated by photons.” Olivia Meyer-Streng
Original publication:
A. Gonzalez-Tudela, C.-L. Hung, D. E. Chang, J. I. Cirac, and H. J. Kimble
Subwavelength vacuum lattices and atom-atom interactions in photonic crystals Nature Photonics, 6 April 2015, Advanced Online Publication
Contact:
Prof. Dr. Ignacio Cirac
Honorary Professor, TU München
Director at the Max Planck Institute of Quantum Optics
Hans-Kopfermann-Straße 1 85748 Garching
Phone: +49 - 89 / 32905 -705/736
Fax: +49 - 89 / 32905 -336
E-mail: ignacio.cirac@mpq.mpg.de
Dr. Alejandro González-Tudela
Max Planck Institute of Quantum Optics,
Hans-Kopfermann-Straße 1 85748 Garching
Phone: +49 89 / 32905 -127
E-mail: alejandro.gonzalez-tudela@mpq.mpg.de